Collaboration Casts New Light On Quantum Dots
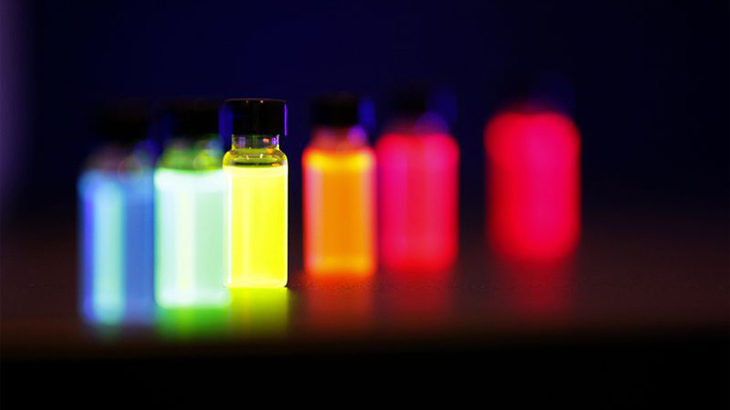
Tiny specks or ‘quantum dots’ of gallium nitride (GaN) – described as the most significant semiconductor material since silicon – could deliver a dramatic leap forward in data processing speeds and data security, thanks to ground-breaking work at Cambridge and Oxford Universities.
As part of a close and fruitful collaboration dating back to 2003, Rachel Oliver, Professor of Materials Science at Cambridge, and Robert Taylor, Professor of Condensed Matter Physics at Oxford, are leading research to help release these dots’ remarkable potential and answer crucial questions about their characteristics and capabilities.
This dovetailing of complementary expertise spanning two SES Consortium members has already delivered a stream of ‘world-first’ breakthroughs in the field of optoelectronics – the branch of electronics that involves the harnessing of light – with the promise of many more to come.
Putting Photons in Focus
What subatomic quarks are to matter, photons are to light. But as well as comprising the smallest amount of light that can exist, they present big scope for game-changing scientific advances. For example, these ‘packets’ of energy can be exceptionally effective data carriers, enabling ultra-secure encryption and improved processing speeds that could help transform computing and communications.
“Pooling our capabilities and facilities is critical to negotiating the route from fundamental understanding to proof-of-concept to basic prototypes and on towards practical devices.”
Rachel Oliver, Professor of Materials Science at Cambridge
But the challenge of unlocking these benefits is also huge –particularly in terms of developing reliable Single Photon Sources that generate separate photons on demand. Rachel Oliver has confronted this challenge throughout her career to date. Her key focus is the potential of GaN-based quantum dots to act as Single Photon Sources.
“Just a few nanometres in diameter, these dots are so small that it’s one of their natural properties to emit one photon at a time,” she explains. “Quantum dots were originally ‘discovered’ in the 1980s. Fabricating them from GaN now offers the opportunity to develop single photon emitters that don’t need cryogenic temperatures in order to function effectively. ”
Working closely in tandem with Professor Taylor’s group at Oxford, Professor Oliver and her colleagues at Cambridge have already delivered a string of achievements, including:
- Development of the first blue-emitting Single Photon Source to utilise quantum dots.
- The first ever measurements of GaN-based dots’ key properties, including the first observations of Rabi oscillations and other quantum phenomena.
- New techniques not just for growing the dots but also for arranging the crystals they grow on – a breakthrough increasing photon emission rates by a factor of 10.
The collaboration’s latest Engineering and Physical Sciences Research Council (EPSRC) funded project continues to extend understanding, with a clear focus on developing crystal growth techniques conducive to incorporating the quantum dots into practical devices.
1. Close-up of part of the Metalorganic Vapour Phase Epitaxy (MOVPE) system used to grow the quantum dots. This section controls the flow of various gases into the reactor, 2. Atomic force microscopy image of non-polar quantum dots (the small white blobs in the image), 3. Dr Tim Puchtler (who was a graduate student in the Oliver group in Cambridge and is now a post-doc in Oxford) working on the Oxford optical characterisation system, 4. Oxford optical setup for measuring quantum dot optical properties
Pioneering Partners
Underpinning this ongoing success story is the exemplary working relationship between Cambridge and Oxford, rooted in Professor Oliver’s time as a PhD student in Oxford between 2001 and 2003. Such links are continually reinforced: one former PhD student from the Cambridge team currently holds a post-doctoral post at Professor Taylor’s laboratory.
“The capabilities and facilities that we each offer are distinctive and complementary”, Rachel Oliver explains. “Pooling them has been critical to negotiating the route from fundamental understanding to proof-of-concept to basic prototypes and on towards the practical Single Photon Sources that are our ultimate goal.”
- The Cambridge team provides outstanding expertise in manufacturing quantum dots and engineering them to deliver the required performance. Facilities include two (soon to become three) industry-standard nitride growth reactors for the chemical vapour deposition method known as Metalorganic Vapour Phase Epitaxy (MOVPE). The university’s Centre for Gallium Nitride is also an official EPSRC research hub.
- The Oxford team provides the skills needed to understand, characterise and exploit nanoscale structures in GaN-based materials, including proven capabilities in examining and testing quantum dots and getting to grips with complex quantum phenomena. For example, highly sophisticated systems are capable of optically distinguishing the dots, measuring and mapping the extremely low levels of light they emit, and revealing spectral, spatial and other properties. The team’s many achievements include measuring electric fields on a single quantum dot.
“A key feature of our collaboration is very effective iteration,” comments Robert Taylor. “As well as high-quality quantum dot samples, our Cambridge colleagues provide excellent data on what these comprise. With rapid turn-round, we can then look at the samples, provide advice on how the materials could be improved, and shed light on how structure, properties and performance are all intrinsically tied together. This input is directly fed back into the quantum dot production process at Cambridge.”
Tackling Quantum Dot Questions
Although still in its first year, the current EPSRC-funded project is already generating fresh insights that are bringing practical GaN-based quantum dot technology closer to commercial viability. Recent breakthroughs include:
- A new way of making ‘mirrors’ that very precisely direct the photons emitted by the quantum dots.
- Integration of the dots into nanostructures delivering improved signal-to-background ratios.
- Single photon emission at temperatures up to 160K.
Of course, many challenges remain – especially the issue of defects. “Unlike most modern semiconductor materials, the nitrides contain very high defect densities and these
“A key feature of our collaboration is very effective iteration – Cambridge provide high-quality samples that we then look at to provide advice on how the materials could be improved”.
Robert Taylor, Professor of Condensed Matter Physics, University of Oxford
influence the properties and performance of devices made from them,” Rachel Oliver explains. “For instance, the defects impact on quantum dots’ ability to emit photons that are indistinguishable in colour. Moving forward, we need either to eliminate the defects or to identify quantum dots that are more resilient to them.”
Initially, the market for photon emitters and detectors made possible by this benchmark Oxford-Cambridge collaboration is likely to centre on other laboratories, and specifically the routine deployment of devices that are affordable and easy to operate. In the longer term, however, there is the real prospect that Professor Oliver and Professor Taylor’s work will help catalyse advances in computing and communications that revolutionise the way we bank, the way we shop – and many other facets of our lives.
Contacts
Project contacts
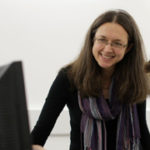
Dr Rachel Oliver
Professor of Materials Science, University of Cambridge
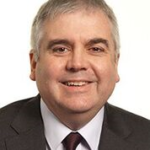
Professor Robert Taylor
Professor of Condensed Matter Physics, University of Oxford
Publications
- Jarjour, A. F., Oliver, R. A., & Taylor, R. A. Nitride-based quantum dots for single photon source applications. Physica Status Solidi (a), 206(11), 2510-2523 (2009). DOI: 10.1002/pssa.200824455
- Jarjour, A. F., Oliver, R. A., & Taylor, R. A. Progress in the optical studies of single InGaN/GaN quantum dots. Philosophical Magazine, 87(13), 2077-2093 (2007). DOI: 10.1080/14786430701311219
- Reid, B.P.L., Kocher, C., Zhu, T., Oehler, F., Chan, C.C.S, Oliver, R.A. & Taylor, R.A. Non-polar InGaN quantum dot emission with crystal-axis oriented linear polarization. Applied Physics Letters, 106, 171108, 2015. DOI: http://dx.doi.org/10.1063/1.4919656
- Reid, B.P.L., Kocher, C., Zhu, T., Oehler, F., Emery, R., Chan, C.C.S, Oliver, R.A. & Taylor, R.A.
- Observations of Rabi oscillations in a non-polar InGaN quantum dot. Applied Physics Letters, 104, 263108, 2014. DOI: dx.doi.org/10.1063/1.4886961
- Zhu, T., Oehler, F., Reid, B.P.L., Emery, R., Taylor, R.A, Kappers, M.J. & Oliver, R.A.
- Non-polar (11-20) InGaN quantum dots with short exciton lifetimes grown by metal-organic vapor phase epitaxy. Applied Physics Letters, 102, 251905, 2013. DOI: dx.doi.org/10.1063/1.4812345
- Jarjour, A. F., Oliver, R. A., Tahraoui, A., Kappers, M.J, Humphreys, C.J & Taylor, R. A. Control of the oscillator strength of the exciton in a single InGaN/GaN quantum dot. Phys. Rev. Lett., 99, 197403, 2007.
- Robinson, J.W., Rice, J.H., Lee, K.H, Na, J.H., Taylor, R.A., Hasko, D.G, Oliver, R.A., Kappers, M.J, Humphreys, C.J & Briggs, G.A.D. Quantum-confined Stark effect in a single InGaN quantum dot under a lateral electric field. Applied Physics Letters, 86, 213104, 2005.
- Rice, J.H., Robinson, J.W., Jarjour, A. F., Taylor, R.A., Oliver, R.A., Briggs, G.A.D, Kappers, M.J & Humphreys, C.J. Temporal variation in photoluminescence from single InGaN quantum dots. Applied Physics Letters, 84, 4110-4112, 2004.