From biodiesel to detergents
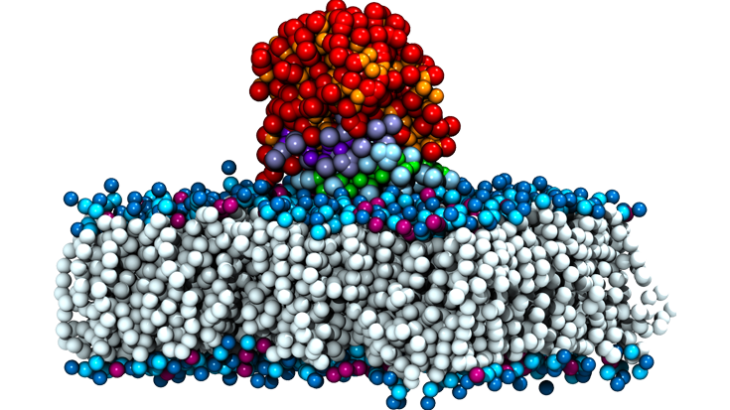
At the University of Oxford, Nathalie Willems is using the Emerald supercomputer in research that could bring about major benefits in a number of industrial areas, leading to processes that are not only more efficient and cost effective but also better for the environment.
Lipases perform a vital role in living organisms, breaking down large molecules of fat, for example, in the human digestive system. Lipase enzymes are used in countless industrial processes, ranging from paper to biodiesel production. They are used in detergents and to improve the quality of drug manufacturing.
Lipases are proteins that act as biological catalysts to break down oils and fats. To increase the efficiency of the industrial processes that lipases work in they are immobilised. This means that the enzyme is trapped on or within a particular surface or interface, which gives it increased stability and in some cases, activity. Immobilisation can enable the enzyme to stay active for a longer period of time while it does its work.
Ascertaining which surfaces work best for immobilisation has the potential to dramatically reduce industry costs, improve efficiency and sustainability. Finding surfaces that are particularly good at holding on to lipases also mean that stocks of these enzymes, which are often costly to produce, do not need replenishing as often. In addition the enzymes can also be re-used, in some cases for a number of years.
At the University of Oxford, PhD student Nathalie Willems is carrying out investigative research in this area using the simulation capability of the Emerald supercomputer. Nathalie is based at the Department of Biochemistry, which is led by Professor Mark Sansom.
Computer simulation of the M37 immobilisation process – showing how the enzyme (the smaller cyan and green region) becomes attached to a surface
Nathalie is concentrating on three particular types of lipase enzyme (M37, CalA and TLL) and is using Emerald to predict how their shape is changed or affected when they interact with different surfaces, and which surfaces lead to a more active protein.
Three very different types of surface are being looked at: cellulose (the most abundant carbohydrate molecule on Earth), a biological membrane and the revolutionary material graphene (with its incredible mechanical strength and electric and heat conductivity). This trio of surface types provides Nathalie with a range of structural properties to investigate, so she can see how the enzyme interacts with all three and also how the shape of the lipase is affected.
- M37 is being studied due to its potential in biodiesel production.
- CalA (Candida antarctica Lipase A) is produced by a yeast that is found in Lake Vanda in Antarctica. However, despite its icy links, it can remain stable at very high temperatures. It is used in production processes in the pulp and paper industry to hydrolyse compounds that melt at very high temperatures.
- TLL (Thermomyces lanuginosus lipase) is used in biodiesel production and the food and detergent industries.
“Lipase enzymes rank quite highly on the expensive scale in terms of industrial production costs. In the computer modelling we are able to examine different surfaces to see which ones do the job better of immobilising, holding and retaining the lipase. With our research Emerald can run powerful software that works like a computational microscope. This software can zoom in on the interactions of proteins in atomic-scale detail. Not only does this computer simulation work provide great insight, but Emerald is also able to do, in a couple of days, what would take a desktop PC months to do.” Nathalie Willems, Department of Biochemistry, University of Oxford
Enzyme attached to a surface
At the moment Nathalie is using Emerald to concentrate on these three specific enzymes. Taking M37 for example, understanding more about this enzyme and optimising its interactions with different surfaces could lead to a much higher yield of product in potential applications such as biodiesel production. Better yields will also lead to less waste in the production process.
‘As we get better at understanding how M37 interacts with different surfaces, we can also propose how to optimise this enzyme in applications such as biodiesel production. As such, we could end up with an enzyme that is more efficient in this context than any other currently known and is also less harmful to the environment”. Nathalie Willems, Department of Biochemistry, University of Oxford
In the long term the same computer simulation research techniques could be broadened out and applied to many more areas around the bioscience challenges of the 21st Century.Nathalie is funded by the Biotechnology and Biological Sciences Research Council (BBSRC) as part of the Interdisciplinary Bioscience Doctoral Training Partnership.
- For more information on Nathalie’s research (Multi-scale molecular dynamics studies of lipase enzymes at different surfaces) see:http://www.bioch.ox.ac.uk/aspsite/index.asp?pageid=599 and http://sbcb.bioch.ox.ac.uk/currentmembers/willems.php
- Emerald: http://www.cfi.ses.ac.uk/emerald/
- University of Oxford, Department of Biochemistry: www.bioch.ox.ac.uk/
- Biotechnology and Biological Sciences Research Council (BBSRC): www.bbsrc.ac.uk