Guest Post: Exploring the Materials Genome
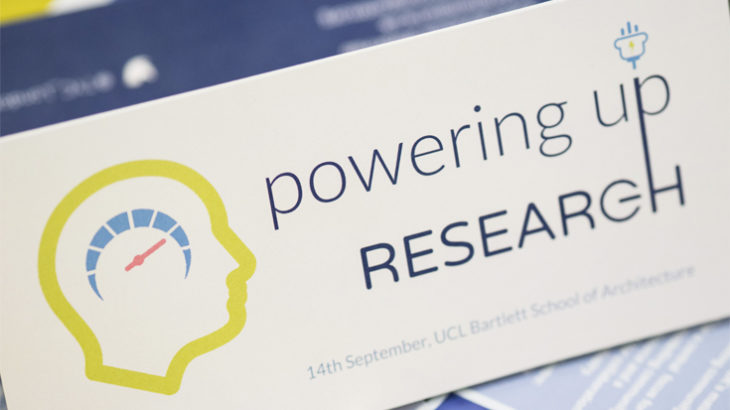
Currently a PhD candidate in the group of Prof. Angelos Michaelides at UCL, Patrick studied Chemistry (MSc, BSc) at the University of Warwick where his research focused on elucidating the quantum nature of biological light harvesting proteins. He also worked in research for DSM Speciality Resins, where he developed bio-renewable alternatives to polymers sourced from fossil fuels. Patrick’s current research focuses on the application of machine learning algorithms to the development of accurate models for use in molecular dynamics simulations of graphene and other carbon-based materials.
It would – I think – be a fair statement to make that our technological progress as a species may be charted through the materials we have made use of. From the very first wooden tools, no more than sticks, through to the stone, bronze and iron ages, to what might many years from now be referred to as the ‘age of silicon’, the various eras of civilisation have been defined by the materials we have had at our disposal.
“Don’t try to reinvent the wheel”, is a piece of sage advice often given out to those trying something new, an adage which belies the fact that the repeated reinvention of the wheel is actually a wonderful benchmark for progress. The very first wheels, from prehistoric Mesopotamia (c. 3500 BCE) were made of stone and used for pottery – it was not until 300 years later that wheels would be used for chariots. Of course, due to stone’s heavy and brittle nature, wheels for such a vehicle would require an altogether different material: wood. Flexible and strong, wood could absorb the shock of impacts without shattering – later, a rim, formed of iron, prevented buckling over time due to changes in temperature and humidity. Perhaps the most iconic era of innovation, the Industrial Revolution, saw an explosive increase in the rate of technological development. Of course, such a period modernisation required another reinvention of the wheel; the alloyed metal wheels of a train are a masterwork of materials science and engineering, they allowed locomotives to travel across the globe. A modern wheel, by comparison, benefits from uncountable reinventions – the tyres may be made of Kevlar composites, the rims from aluminium or titanium alloys and the overall shape, the tread, thickness and weight will have been extensively optimised to maximise performance. All of this really begs the question, “What’s next, what will the materials of tomorrow look like?”
“For a researcher to achieve expertise on any single length scale (electronic, atomistic or continuum scales) is a challenging enough task, let alone the task of mastering many of them simultaneously”
Prof. Mark Miodownick, from University College London, asked precisely this question during his keynote lecture at last month’s “Powering up Research” conference, which launched both “Thomas,” a new tier two supercomputing facility and the associated Materials and Molecular Modelling Hub. Much as the information age – the age of silicon – has revolutionised our day-to-day lives, so too can we expect it to revolutionise science, in particular materials science. This expectation is recognised not just through Thomas’ dedication to computational researchers in the field of materials science, but through numerous other global programs such as the US’s Material’s Genome Initiative. Computation alone, however, is just a tool, in order to answer the question posed above, we must take a closer look at how these computational resources are put to use by modern materials scientists.As is well known, the properties of a material are governed not just by what elements make it up, but by their arrangement in space. A prime example of this is carbon and its various allotropes. By simply arranging its atoms in a hexagonal pattern we can form graphene (which is 200 times stronger than steel), if instead we position these atoms tetrahedrally, we produce diamond. By arranging 60 atoms of carbon into the football-like shape of a Buckminster-Fullerene, we find ourselves with an efficient electron acceptor for modern solar cells. Prof. Chris Pickard (Cambridge) made the excellent point at the Powering up Research conference, that there are many, many, billions of possible such arrangements of carbon – how could we possibly search through them all? The short answer is, we don’t. It is an interesting quirk of the energy landscape of chemical structures that the most stable ones are often the easiest to find – this allowed Pickard to use the aptly named ‘Ab Initio Random Structure Search’ to find candidate materials. The method (semi-)randomly places atoms in space, before allowing the laws of quantum mechanics to dictate the most stable arrangement for these to take. In doing so, he has uncovered a vast menagerie of possible crystal structures for various materials, many of which had not yet been experimentally uncovered and many of which show promising properties for a number of applications.Of course, one could never explore all possible combinations of all possible atoms, it is famously said that the elements C, H, O and N alone could combine to make 1060 unique compounds with a mass less than 500 grams per mole. One way to use the results of experiments like Pickard’s to systematically explore ‘chemical space’ would be to use the vast library of possible materials generated experimentally and theoretically to begin to map out a so-called ‘materials genome’, a roadmap of sorts, designed to show which atomic level changes combine to produce a given change in the final material. If future researchers required a new malleable polymer with strong UV resistance for the wheels of the next Mars Rover, such aroadmap might be able to provide them with strong initial candidate materials. This ‘materials genome’ is arguably the holy grail of materials science and is undoubtedly still many decades from being realised, however, progress is already being made in this direction through the application of machine learning algorithms, a topic which is relevant to my own research. In the information age, machine learning is rapidly emerging as an indispensable tool – Silicon Valley giants Google, Microsoft and Facebook rely heavily on these algorithms to sort through and analyse petabytes of data. Based on this analysis, these algorithms can make accurate predictions, which often elude their human counterparts. The application of these same algorithms to materials science is one possible way that has been identified in which we may be able to use computing to improve our predictive capabilities. I consider this to be a particularly exciting development; machine learning can only be successful given sufficient ‘training data’. In materials science, the generation of meaningful training data – realistic structures and associated properties – is highly challenging. A connection between methods for the rapid generation of candidate structures and machine learning methodologies to learn from their properties and make predictions would certainly be intriguing.A theme that was present through many of the day’s talks, in particular that of Prof. Daniele Dini, was the nature of materials as inherently multi-scale objects. Much as the arrangement of atoms in space can determine the properties of carbon, so too can the macroscopic construction of a material also have a powerful impact on how it behaves. Dini discussed how it is possible to link the behaviour at differing length scales – from the electrons in an atom, to entire ‘objects’ such as the bearings, which allow a vehicle’s wheels to spin freely. The importance of the multiscale nature of materials design is particularly prevalent in the development of meta-materials and composites, whereby a particular arrangement and combination of many different materials can result in a final product with properties, which are superior to its component parts. Multiscale modelling is a discipline thatcan be expected to benefit hugely from an organisation like the Materials and Molecular Modelling Hub (and associated computational resources). For a researcher to achieve expertise on any single length scale (electronic, atomistic or continuum scales) is a challenging enough task, let alone the task of mastering many of them simultaneously. The MMM will facilitate research across groups which might not otherwise come into contact, thereby promoting the development of materials which have truly been designed ‘from the ground, up’.It was clear from this meeting that materials science is a field, which shows no sign of slowing down. As society demands more from its technology, so too will it demand more from its materials. Computation is set to continue to have a radical impact on how new materials are discovered and put to use – something that the investment in the Thomas supercomputer recognises. As for what materials the wheels of the future might be made of, we still don’t have an answer for Prof. Miodownick’s question. We might, however, take a guess that they will be found first on silicon chips, as digital representations of materials rather than as the materials themselves.